X-Ray Diffraction (XRD) is a technique for examining the arrangement of atoms in a crystal lattice. This is a comparative technique where the pattern obtained for a sample is compared against standards collected over the hundred year history of the technique. Elemental composition is not determined by this technique but can be inferred from the results. Confidence in matching the phases can vary depending on how closely the database pattern matches the fraction present in the sample, the number and type of other phases present in the sample, crystallinity, concentration of the phase and the quality of the XRD trace.
Other information that can be gleaned from an XRD trace includes the degree of weathering or alteration, crystallite size, substitution, degree of disorder and the amorphous content.
In real world samples it is common to have over a dozen different crystal phases at varying concentrations in a complex sample. The use of elemental assay, information of the samples history and location and complimentary techniques all assist by strengthening confidence in making phase identification.
XRD techniques vary from qualitative to semi quantitative through to full Quantitative Rietveld analysis. Each technique will deliver different levels of accuracy and precision proportional to the amount of work required to improve accuracy through sample preparation, instrument running and analysis.
One of the most challenging areas for phase identification is clay speciation. Due to the poor crystallinity and irregular ordering of some clay groups, identification may require further work involving glycolation, heat and other techniques.
At Microanalysis we have several automated powder X-ray Diffractometers. We have a number of diffraction databases including the latest 2018 complete
International Centre for Diffraction Database (ICDD). Our standard holders take approximately one cubic centimetre of powdered sample. We also have holders that take milligram quantities of sample, holders that can present filter cloth, flat ceramic or metallic plates and holders that can accommodate some sample irregularity.
The techniques we frequently utilise are:
- Qualitative XRD where the phase identification is conducted and the minerals are classified as major, minor and trace.
- Semi-quantitativein which the phase identification includes a concentration which is calculated using the normalized reference intensity ratio method where the intensity of the 100% peak divided by the published I/Ic value for each mineral phase is summed and the relative percentages of each phase calculated based on the relative contribution to the sum. An estimation of the amorphous content can also be given.
- Spiked semi-quantitative which is similar to a standard semi quant with a higher confidence in the concentration values and the estimate of the amorphous content.
- Quantitative XRD analysis using an internal standard, with Rietveld analysis to give the highest confidence possible in the concentration of phases and amorphous content determined.
- Crystalline silica determinations for alpha quartz, cristobalite and tridymite. A full phase identification is performed to check for overlaps that may be present from phases present. A specific peak scan is then performed with this result compared against a five point calibration curve to produce a value of each crystalline silica phase present.
- Clay speciation. A Semi-quantitative analysis is performed followed by gylcolation and heating to determine specific clay groups.
- Respirable free silica determination. A semi-quantitative analysis is performed on the respirable fraction and is combined with particle size determination and is checked using scanning electron microscopy.
- Comparative XRD. If there is no entry in the databases for the compound you examining but you’re have or can construct a suitable reference pattern standard, then this technique would still be of value to you.
- Specific mineral semi-quantitative analysis. The phase(s) of interest are concentrated through solvent washing, heavy liquid separation, magnetic separation or other specific techniques to give better confidence in the identification or to enhance XRD features to study substitution.
Microanalysis is always pleased to customise XRD techniques to accommodation specific requirements and samples to achieve the highest quality results to meet the price point of the client.
Ian Davies – Analytical Scientist
Speciating contaminants in everyday products, from food and beverages to crime scenes, from building materials to aviation fuel, understanding the composition of errant particles is key to determining the source and solving the reason they ended up in places they weren’t meant to be! Microanalysis Australia is keen to announce the addition of an FTIR microscope to it’s line of investigative equipment.
Our PerkinElmer Spectrum2 now has an accessory which allows enhanced spatial resolution to discriminate particles down to 20 µm in size against backgrounds which would otherwise swamp a typical ATR measurement. Multi-layer specimens such as paint films can now be analysed to determine polymer composition differences between coatings – matching paint flecks to potential sources and confirming proper application etc, to name a few. Microanalysis is routinely requested to filter solutions, from potable waters, to agrochemicals to look at known contaminants and determine possible sources. Whilst SEM/EDS has been an enormous help with these unknowns in the past, FTIR microscopy adds another string to the bow of being more absolute in pin-pointing the ultimate source of the tinniest of particles.
On Wednesday 1st November, Microanalysis held a special information evening for the Australasian Corrosion Association (ACA) and Surface Coating Association Australia (SCAA) professional associations.
Pulling everyone away from the tasty nibbles and drinks, the 12 members had a guided tour of the Laboratory to learn a little of what Microanalysis Australia is up to in the corrosion field as well as in a diverse range of other industry sectors.
Exploring the lab, the delegates learned about spatial elemental analysis in the two Zeiss SEM/EDS systems, identifying corrosion products versus naturally occuring ferrous phases in the two Philips/PANAlytical XRDs, sizing microbes in the Malvern Mastersizer, understanding settling rates using a Sedigraph, Hiac Royco Particle Counters for contaminat concentration determination in liquids – particularly important for corrosion inhibitor cleanliness and surface corrosion and pitting analysis on the Solarius Laser Profilometer.
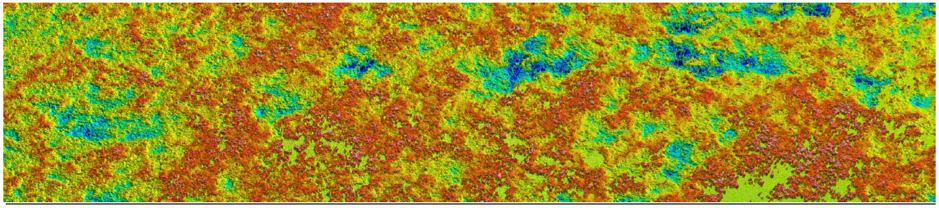
The group learned about the UNDG testing that Curtin University and Microanalysis have been involved in with regards the C1 ‘Localised Corrosion – Intrusion depth’ research. The Solarius Profilometer has been an invaluable tool in terms of scanning large corroded areas to obtain pit depth and profiles.
After the tour, the group returned to the drinks and food where Nimue Pendragon and Owen Carpenter presented some information about failure analysis using SEM EDS, bulk goods testing by ADG and IMDG, and UNDG C1 corrosion analysis by laser profilometer. Download a copy of the presentation below.
We hope an informative evening was had by all.